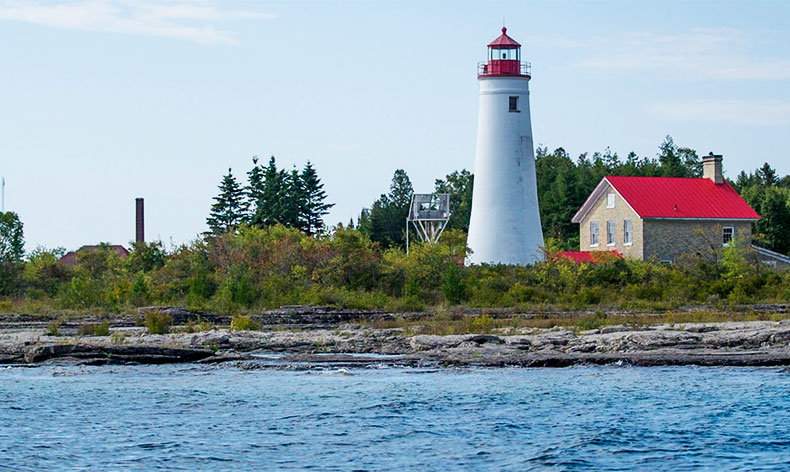
Pressures on the Sanctuary
Natural processes and human activities threaten the long-term sustainability of the Thunder Bay sanctuary's shipwrecks and other maritime archaeological resources. Natural processes such as ice, waves and aquatic invasive species such as zebra and quagga mussels have both known and potential negative impacts on maritime archaeological resources. Human activities have the greatest potential for damaging sanctuary resources. They include anchoring, inadvertent and intentional diving practices that damage resources, and looting. While encouraging increased public access to the unique and irreplaceable shipwrecks of Thunder Bay, the sanctuary strives to balance increased visitation with resource management and preservation. This section describes the nature and extent of the most prominent pressures on Thunder Bay National Marine Sanctuary resources.
Diving and Research
Due to the large number of easily accessible shipwrecks, the area in and around the sanctuary is a popular snorkeling and diving destination. Visiting dive boats and divers have the greatest potential to negatively impact the quality of sanctuary resources. This includes anchor damage to shipwreck sites, leaving temporary mooring lines attached to sites which later become derelict, poor diving practices by divers (e.g., brushing mussels off delicate wood surfaces or handling, moving or inadvertently damaging artifacts), and souvenir hunting and looting (Figures 30 and 31). Substantial damage can occur from anchors, whereby a visiting dive boat "hooks" a shipwreck to both locate the site and secure the boat's anchor.

Due to advances in mixed-gas "technical" and closed-circuit rebreather diving there have been an increased number of divers at deepwater shipwreck sites (130- to 300-foot depths) over the last decade. Generally, shipwrecks at these depths are more intact that shallower sites, due to a less dynamic environment. These sites also possess a greater potential for artifacts to survive, due to the limited - though increasing - number of visitors. Given this, the impacts of looting and anchor damage are relatively greater at these sites. Moreover, new shipwreck discoveries at deeper depths continue to occur. Local shipwreck hunters and divers continue to find remarkably well-preserved shipwrecks at these depths, as does the sanctuary and its partners, who discovered five shipwrecks between 2002 and 2011. Negative impacts from diving at these sites are the same as described above, with potentially greater consequences given that the sites are generally more intact (often with fragile features preserved) and possess a greater number and variety of artifacts (Figures 32-34).


Non-indigenous Species
Since the 1800s, human activities have caused the introduction of more than 200 exotic aquatic organisms of all types, including plants, fish, algae and mollusks, in the Great Lakes (Great Lakes Commission 1992, 2007). These species have the potential to cause devastating and often permanent damage to the Great Lakes ecosystem by degrading beaches and swimming areas, changing water quality and clarity, competing with native species for food and habitat, and altering complex food webs that support the aquatic ecosystem (Great Lakes Commission 2007). Of the many invasive species in the Great Lakes, zebra and quagga mussels have had the greatest negative impact on sanctuary resources (Figure 35). Additionally, other invasives that degrade water quality have an indirect effect by potentially limiting physical access to sanctuary resources (e.g., a beach closure that prevents snorkeling or diving at a near-shore site).
Zebra mussels (Dreissena polymorpha) are small, non-indigenous mussels that are native to the Black, Caspian, and Azov seas. However, most likely due to construction of canal systems, they became widespread throughout Europe by the early 19th century. Zebra mussels were first reported in North America in 1988 in the Great Lakes, but likely became established in 1986. The mussels were likely introduced via ballast water of one or more transoceanic ships. Populations first became abundant in Lake St. Clair and western Lake Erie and then spread quickly to all the Great Lakes via intra-basin ballast water exchange and other vectors. Zebra mussels are a very successful invader - they live and feed in many different aquatic habitats, breed prolifically, and have a planktonic larva stage that allows them to drift with currents and spread rapidly. By the early 1990s,

Quagga mussels (Dreissena bugensis), a close relative of the zebra mussel, are native to the northern portion of the Black Sea. Similar to zebra mussels, their range expanded with the construction of canals throughout Europe. They were first observed in Lake Erie in 1989 and by 2005 had become established throughout the Great Lakes. Like zebra mussels, quagga mussels were most likely introduced into the Great Lakes region from ballast water discharge of transoceanic ships. While zebra mussels are mostly confined to hard objects and substrates, quagga mussels are far more versatile. Unlike zebra mussels, quagga mussels can also live and thrive directly on muddy or sandy bottoms. In addition, quagga mussels can tolerate a wider range of temperatures and water depths than zebra mussels. In particular, they are also highly prolific and capable of spawning in deeper regions with continuously cold temperatures (MSG 2009b).

Zebra and quagga mussels directly and indirectly affect natural ecosystem functions by altering nutrient cycles and re-engineering physical habitat. Their greatest direct impact is caused by their feeding habits (Vanderploeg et al. 2002, Nalepa et al. 2007, MSG 2009a). They are voracious filter feeders; each mussel is capable of filtering one liter of water per day and removing vast amounts of phytoplankton and suspended particles from the water, thereby depleting the food supply for many native invertebrates found in both the water (zooplankton) and bottom sediments (benthic macroinvertebrates). As a result, these organisms decline in numbers, which in turn has a severe impact on the many fish species that depend on them as a food source. Zebra and quagga mussels attach themselves to any hard surfaces, which includes the shells of native mussels, or unionids. This behavior, called bio-fouling, has greatly reduced populations of native mussels and some other sessile organisms (Gonzalez and Downing 1999, Bially and Macisaac 2000, Nalepa et al. 2007, MSG 2009a). These mussel "reefs" can also dramatically alter water flow regimes by changing the roughness and slope of the benthic habitats.
Zebra and quagga mussels have an affinity for hard surfaces such as boat hulls, engines, docks, buoys, breakwalls, pipelines and submerged archaeological resources (Watzin et al. 2001, MSG 2009a). Therefore, they are a significant nuisance for municipal water treatment plants and power plants. Zebra mussels have the ability to clog water intake valves that are used in pumping stations, electric generating plants, and industrial facilities. Between 1989 and 2004, the cost to control mussels at municipal water plants and power plants in the Great Lakes was about $18 million per year (Connelly et. al., 2007).
The presence of invasive mussels on shipwrecks and other submerged maritime archaeological sites constitutes a significant challenge for archaeologists, historians and resource managers, and in many cases reduces a site's archaeological integrity. In terms of detailed archaeological documentation, the baseline has shifted in regards to the quality of data that can be obtained (at least easily) from shipwrecks sites. For example, a timber, feature, or artifact that is encrusted with several inches of mussels will not yield an accurate measurement without removing the covering mussels, an activity that poses its own challenges and is difficult to do on an entire shipwreck site, or even for a representative number of features (Figures 37 and 38).

Research on shipwrecks in Lake Champlain, which has cold, fresh water similar to the Great Lakes, strongly suggests that zebra mussels are accelerating the corrosion of iron fasteners and features of shipwrecks there, probably due to the complex communities of bacteria that accumulate under the thick layers of mussels. Additionally, water quality data definitively document the loss of iron into the water column above the surface of zebra mussel colonies on the wrecks (Watzin et al. 2001). Although the long-term implications of the findings on the structural integrity of shipwrecks is still not clear, it is reasonable to expect that some degree of physical integrity is being lost. Of additional concern is the weight that multiple layers of mussels add to wrecks sites, particularly to pieces of the wreck that may already be loosely attached or breaking off. Moreover, the total weight of mussels encrusted across an entire shipwreck site can potentially be significant. The weight of mussels has been known to sink submerged buoys, and similar forces are surely at play on shipwreck sites. Notably, shipwrecks in depths where limited sunlight and colder water results in less available food were once thought to be beyond the reach of invasive mussels, though since approximately 2003, these sites too have been colonized by quagga mussels.
The public interpretation of shipwreck sites, and perhaps even the public's appreciation for shipwrecks, is also challenged by the presence of thick layers of detail-obscuring mussels. Interestingly, filter feeding mussels have greatly improved water clarity throughout the Great Lakes, presenting a Faustian bargain of sorts. Throughout the Great Lakes, water clarity has increased by 38.5 percent (Higgins and Vander Zander 2010). According to studies by NOAA's Great Lakes Environmental Lab in offshore Lake Michigan, secchi depth7 was 19.7 feet in 1985-1989, 23 feet in 1995-1998, and 59 feet in 2007-2010. Although quagga mussel numbers are higher in Lake Michigan than Lake Huron, these values give an estimate of the impact mussels can have on water clarity (T. Nalepa, NOAA GLERL, pers. comm., 2012). With quagga mussels now displacing zebra mussels in much of Lake Huron, a similar trend can be expected in and around the sanctuary. Today in Lake Huron, there are seasonal periods where visibility can reach 100 feet, and average visibility in offshore areas is 50 feet or better throughout the year. Prior to invasive mussels in the Lake Huron, visibility rarely exceeded 20 feet and was often less. Consequently, divers, snorkelers, kayakers and tourists aboard glass bottom boats now have a far easier time seeing shipwrecks, albeit they are colonized by invasive mussels.
Natural Deterioration
Shipwreck sites naturally deteriorate over time, and the speed at which deterioration occurs is determined by environmental conditions. The presence of salt versus fresh water, certain marine organisms, warm versus cold water, bottom sediment type, water depth, the potential for ice formation, and other local geographical and environmental factors all contribute to the natural deterioration of shipwrecks. As mentioned previously, the cold, freshwater environment of Lake Huron helps preserve sanctuary shipwrecks by inhibiting marine organisms harmful to shipwrecks and slowing corrosion that occurs in marine environments. However, ice movement, currents, shifting sediments, storms and normal wave action are all environmental factors that can be detrimental to shipwrecks, particularly those in shallow depths (Figures 39 - 42).


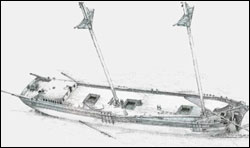
Individual artifacts may survive well in both shallow and deep-water environments. At shallower sites with loose sediment, smaller artifacts may be dispersed, thus losing their archaeological context; however, they can also become physically protected by being buried in the lake bottom. Buried artifacts can also benefit from a low-oxygen environment, which slows deterioration. Shallow sites located on cobble or hard bottom understandably have a lesser chance of being preserved in sediment, though here too some artifacts will survive. At deeper depths, artifacts typically have a higher likelihood of surviving in their original positions, offering a clearer context. It is not uncommon for an entire complement of deck hardware (windlass, pumps, winches, etc.) to remain fixed in their original positions, or for personal effects and smaller artifacts to remain in localized areas such as a deck cabin. Here too, fresh water helps preserve artifacts, even those not buried in protective sediments. Paper, leather, bone and other organic artifacts can survive remarkably well in fresh water. Glass, ceramic, wooden and metal artifacts are often found in excellent states of preservation. Many of these types of materials occur in and around the sanctuary.
There are potential links between climate change in the Great Lakes and the deterioration of shipwrecks, but research has not been done on the topic, and questions remain. For example, should climate change result in significantly decreased ice coverage on the Great Lakes, this would enhance evaporation and lead to a water level decline. As water levels drop, shallow water shipwrecks may become more exposed to air, waves and ice, thus accelerating natural decomposition. Nearshore shipwrecks in sandy lakebed environments may suffer increased deterioration as increasingly mobile sediment (due to a more dynamic environment created by lower water levels) variously exposes and buries sites. Moreover, the sudden occurrence of a shallow-water shipwreck exposed by shifting sediment makes for an exciting discovery, but one that is also potentially very accessible, and can lead to both intentional and unintentional human impacts. The effects of climate change on a delicately balanced and already stressed ecosystem could also have negative impacts by reducing water quality and, consequently, public accessibility to sanctuary resources.
Fishing and Boating
The primary groups using the Lake Huron fisheries are state licensed commercial fishers, recreational anglers, and Native American commercial fishers. Commercial fishing has declined significantly since the 1940s, when commercial fish stocks collapsed. In 1930, close to 7,000 people were employed in the commercial fishing industry, but by 1975, a little over 1,100 people were employed. Since 2001, only two state-licensed and two to four tribally licensed commercial fishing operations have been operating out of Alpena County. Gillnets are no longer permitted for commercial fishing in central and southern Lake Huron, including within the sanctuary boundaries; trapnets are the only gear used. Today, the Thunder Bay region of Lake Huron is considered one of the most lucrative whitefish fishing grounds in the Great Lakes, and whitefish is the principal commercially harvested species within the Thunder Bay region (NOAA 1999). In 2000, about 60% of all lake whitefish came from Lake Huron (Kinnunen 2003). This decrease in commercial fishing has led to less impact from fishing gear at shipwreck sites.

Commercial and recreational fishing and boating are potential stressors to sanctuary maritime archaeological resources, with the biggest threat being damage resulting from deploying, dragging and recovering anchors and nets (Figure 44). Although impacts from fishing lures from trolling is possible (e.g., drifting and anchored fishing boats can become snagged in wrecks sites and potentially damage a fragile site), the potential impact is slight. Derelict lines and lures pose a potential hazard to scuba divers. Although gillnet remnants are known to exist at a couple shipwreck sites in the sanctuary, the future threat is not great given the limited number of commercial fishers in the area and the prohibition of gillnets in U.S. waters of Lake Huron south of Hammond Bay. In addition, the few remaining Native American and commercial fishermen avoid known wreck sites, as they are hazards to fishing gear.
Pressures on Water Quality
While the Lake Huron watershed is home to about 2.5 million people, both sides of Lake Huron have relatively low human population densities. The Lake Huron basin contains no major metropolitan areas. The largest urban centers in the basin are Sudbury and Sault Ste. Marie on the Ontario side and Flint, Saginaw and Bay City on the Michigan side. With populations under 120,000, these urban areas are relatively small compared to urban areas in the more populous Great Lake basins (EPA 2008).
Nonpoint Sources of Pollution
Unlike point source pollution8, nonpoint source pollution comes from many diffuse sources. Nonpoint source water pollution is usually due to rainfall moving over and through the ground and carrying various chemicals. As the runoff moves, it picks up and carries away pollutants, finally depositing them into surface and subsurface (groundwater) waters. Pollutants and contaminants include excess fertilizers, herbicides and insecticides from agricultural lands and residential areas; oil, grease and toxic chemicals from urban runoff and energy production; sediment from improperly managed construction sites and dredging operations; bacteria and nutrients from birds and other wildlife; pet wastes; and faulty septic systems. Eutrophication (an outcome of excess nutrients in the water, such as fertilizers) of nearshore waters has been an ongoing, documented problem in some nearshore waters of Lake Huron. The process of eutrophication has the potential to shift primary productivity from the slower-growing flora (e.g., grasses) to faster-growing species (e.g., algae) (Fourqurean et al. 2003, Wagner et al. 2008). While the eutrophication rate from the watershed is apparently very low, dreissenid mussels have concentrated the lake's nutrients in the nearshore zone and on the lake bottom (Hecke et al). This has favored colonial, benthic algae such as Cladophora, which can tap into these benthic resources and are not eaten by dreissenids. Blue-green algae (cyanobacteria) are another beneficiary of this nearshore shunt of nutrients. Most blue-green algae are also not edible by dreissenids. These changes have actually increased water clarity during most months, except when blue-green blooms occur.Harmful Algal Blooms
A harmful algal bloom (HAB) can occur when certain types of microscopic algae grow quickly in water, forming visible patches that may harm the health of the environment, plants, or animals. HABs are attributed to two primary factors: natural processes such as warm water and poor water circulation and flow, and anthropogenic causes such as nutrient loading leading to eutrophication. These processes can result in large amounts of certain types of phytoplankton (e.g., blue-green algae) accumulating in the water. Aggregations of these organisms can discolor the water, thus making shipwrecks less visible. In addition, some HAB-causing algae can release toxins into the water that adversely impact aquatic organisms and humans. Impacts include fish kills and skin and respiratory problems in humans. HABs have occurred in the waters of almost every U.S. coastal state. Over the last several decades, HABs have caused more than $1 billion in economic losses in the U.S. due to closures of shellfish beds and coastal fisheries, detrimental impacts on tourism and service industry revenues, and public illnesses (Abbott et al. 2009). Data suggests that HABs are increasing in frequency within the last couple of decades (Harvell et al. 1999). Beach closures due to HABs can impact the public's access to sanctuary resources.Beach Closures
Runoff and spills have periodically resulted in high levels of Escherichia coli (E. coli) bacteria in the sanctuary, resulting in beach closures. E. coli is often used as an indicator organism in nearshore water quality monitoring, and while it may not always cause diseases in humans, its presence can indicate that water may be contaminated with organisms that cause human health impacts such as fever, flu-like symptoms, ear infection, respiratory illness, rashes, gastroenteritis, cryptosporidiosis, and hepatitis. Sources of polluted and contaminated water include runoff from urban, suburban and rural areas, aging sewer infrastructure systems pressed to meet increasing demands, and contaminated flows from other upland sources. Contributing factors that generate these sources include illicit storm drain connections, improper disposal of materials or maintenance that clog pipes and cause overflows, cracked or damaged pipes, overflow of sewer systems during storm events, septic system leaching, and various domestic and wildlife sources.. . . . . . . . . . . . . . .
6The NOAA Office of National Marine Sanctuaries supports the Annex Rules of the UNESCO Convention on the Protection of the Underwater Cultural Heritage (2001). The Annex Rules are a detailed set of guidelines for managing activities directed at underwater cultural heritage based on the International Council on Monuments and Sites Charter on the Protection and Management of Underwater Cultural Heritage (1996). The Annex Rules outline basic principles for the practice of responsible underwater archaeology and provide specific guidance for research, documentation, and artifact curation.
7Secchi depth measurements are used to determine water transparency and clarity.
8Point source pollution results when a pollutant is discharged directly into surface waters from a definite location, such as the pipes of industrial waste facilities or domestic sewage treatment plants.