Authors:
Ian M. Miller1, Caitlin Shishido2, Liam Antrim3, and C. Edward Bowlby3, Editors
1Washington Sea Grant, Olympic Peninsula Field Office, Port Angeles, WA
2Washington Sea Grant, Seattle, WA
3Olympic Coast National Marine Sanctuary, Port Angeles, WA
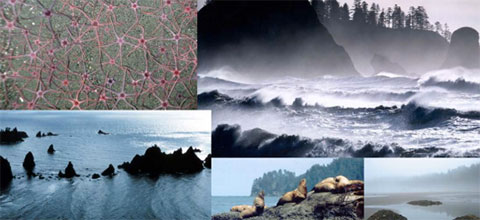
Due to global climate change, the Intergovernmental Panel on Climate Change (IPCC) projects a high likelihood that marine ecosystems around the globe will be measurably altered in the coming century (Bernstein et al. 2007). In some cases, the collapse of entire ecosystems is viewed as possible, or even likely. These projections are valuable in terms of describing the global implications of climate change and clarifying the role that anthropogenic emission of greenhouse gases plays in large-scale ecosystem change. However, they are less useful for assisting managers and policy-makers at the regional or local scale in their efforts to prepare for and, where possible, adapt to climate-related changes.
The Olympic Coast National Marine Sanctuary (OCNMS) is comprised of 8,572 square kilometers of marine and near-shore waters and intertidal habitat off of Washington State's Pacific Ocean coast. As one of 14 national marine sanctuaries managed by the National Oceanic and Atmospheric Administration (NOAA), OCNMS is provided protected status because of extraordinary ecological and maritime heritage values. The Office of National Marine Sanctuaries developed its "Climate-Smart Sanctuary" program in order to facilitate the process of climate adaptation in these special marine waters. This assessment is designed to assist OCNMS in adapting to climate change by bridging the gap between the global projections provided by the IPCC, and the regional and local implications of climate change by 2100.
The direct consequences of climate change on the physical environment in OCNMS were considered and, where possible, the direction and magnitude of change was estimated (Section 2). These physical effects were divided into seven categories: Increasing ocean temperature, ocean acidification, sea level rise, increasing storminess, changing ocean current patterns (with a focus on upwelling), increasing hypoxia or anoxia and altered hydrology in rivers draining into OCNMS. These are summarized here:
- Based on the literature reviewed for this assessment it is considered likely that Pacific Northwest (including the habitats within OCNMS) average air and ocean temperatures will rise measurably by 2100, probably outside of the contemporary range of variability. Downscaling of multiple global climate models for the Pacific NorthWest Coastal zone suggests that ocean water will warm on average, by 1.2°C by 2050, given a range of emissions scenarios (Section 2.2).
- The magnitude and extent of corrosive ocean water (with pH reduced relative to contemporary values and reduced availability of carbonate ions) is also expected to increase. Corrosive ocean water is currently associated with deeper water in OCNMS and is probably only drawn to the surface during periods of intense upwelling. By 2050, however, shallower areas within OCNMS will be exposed to corrosive water with greater frequency (Section 2.3).
- Due primarily to the warming of the ocean and melting of land-based ice, mean true sea level rise in OCNMS could exceed 1.0 m by 2100, but variable rates of vertical land movement associated with tectonic forces will cause variations in the rate of relative sea level rise. Relative sea level is expected to be greater along the southern coast of shoreline within OCNMS as compared to the northern coast (Section 2.4).
- Climate model projections suggest that the tracks of storms in the northeast Pacific Ocean will migrate, on average, further north due to climate change, but it is not clear if the magnitude or duration of storms will change. Observational evidence from locations in the northeast Pacific Ocean suggests the possibility that the ocean adjacent to OCNMS has become stormier in the last 50 years, though it isn't clear if the trend is related to long-term climate trends (Section 2.5).
- Projections regarding the possibility of increased upwelling favorable winds in OCNMS are mixed, and based on the contemporary variability in the timing, duration and intensity of upwelling favorable winds it is considered unlikely that climate change will cause measurable changes by 2100 (Section 2.6). Other factors besides upwelling favorable wind, notably warming of the surface layer of the ocean (Section 2.2), may also influence the timing and magnitude of upwelling and, by extension, productivity in OCNMS.
- Concentrations of dissolved oxygen in the northeast Pacific Ocean are expected to decrease as the upper ocean warms and becomes more stratified. Based on the dynamics of water masses influencing ocean areas within OCNMS, dissolved oxygen concentrations in the ocean waters in OCNMS should be approximately analogous. Long-term declines in dissolved oxygen have been observed at numerous locations in the northeast Pacific, including coastal locations near OCNMS (Section 2.7).
- Future warming in the Pacific Northwest region of the United States is projected to alter regional rainfall patterns and trigger more 100-year magnitude floods and lower summertime low flows among some basins that drain to OCNMS, including the Sol Duc, the Hoh, the Queets and the Quinault Rivers (Section 2.8).
The ecological implications of these likely or possible changes to the physical environment in OCNMS were analyzed in three different ways. First, the general ecological consequences of the suite of changes expected in OCNMS were examined (Section 3.2). These focused on the various ways in which marine ecological systems respond to change - by shifting species' ranges or by altering the timing of life history stages (phenology) for example - or on the consequences of these responses, which include changes in the composition of biological communities in OCNMS and increasing interactions between native" and "non-native species. Given the magnitude of projected climate-related change and evidence from distant and neighboring marine ecosystems it is likely that the marine ecosystem in OCNMS will experience all of these responses or consequences of climate change by 2100. There is some empirical, quantitative evidence from the Northeast Pacific Ocean that some of these responses or consequences are likely already influencing communities in OCNMS (Section 3.2).
Next, the cumulative impacts of multiple changes to the physical environment were assessed for four broad habitat categories: Nearshore and shallow water; deep-sea benthic; pelagic; and freshwater habitats. All habitat categories were vulnerable to various aspects of climate-related change. Chemical-biological impacts due to the changing properties of ocean water within OCNMS (i.e., increased ocean temperature, decreased oxygen concentration, increased acidity) are likely to interact directly with biota in all habitats. By contrast, the "mechanical" consequences of climate change, related to higher or lower streamflow, sea level rise, larger waves, or changes in the magnitude, intensity or location of storms are likely to influence freshwater and nearshore and shallow water habitats most directly. In all cases, though, there was no clear trend related to these changes due to the likely interaction between complex physical and biological systems. For example, in pelagic habitats primary productivity would be expected to increase under climate change scenarios involving increasing upwelling favorable winds and higher CO2 concentrations in ocean water, but rising ocean temperature would be expected to partly or entirely counteract that response by increasing stratification and reducing mixing in the shallow surface ocean.
Finally, the implication of climate-related changes on a select group of species or species assemblages, including phytoplankton, zooplankton, deepsea corals, intertidal mussels, sea urchins (Stronglyocentrotus spp.), Dungeness crab (Metacarcinus magister, formerly Cancer magister), fish, seabirds, cetaceans, pinnipeds and sea otters, was analyzed. There were clear implications of climate-related changes for all species analyzed. In some cases, those implications are due to the direct interaction between the organism and the changing environment, as is the case for some deep-sea coral species whose ability to maintain calcium carbonate body structures may be compromised by ocean acidification (Section 4.4). In other instances, the implications of climate-related change are via ecological interactions that mediate projected changes in the physical environments within OCNMS through other species. For example, given their role as mid- to upper-trophic level predators, the susceptibility of seabirds to climate-mediated changes will depend in large part on the impacts that their prey species experience (Section 4.9). In some instances, the documented consequences of climate cycles (Section 6.3) in the northeast Pacific Ocean are used to suggest the overall impact of climate change on particular species. For example, decreased ocean survival of Chinook and Coho salmon is likely based on observations made during conditions of unusually high water temperatures and reduced or delayed upwelling (Section 4.8).
Despite these very clear implications of climate change, very little certainty could be ascribed to the magnitude or direction of change for a given species or species assemblage, or to the overall consequence of ecological change. For example, despite the clear physical consequences of ocean acidification on some species of deep-sea corals, some capacity to adapt to corrosive ocean water has been demonstrated for species in OCNMS (Section 4.4). In some cases, possible or likely species alterations may not have clear ecological consequences. For example, resident forage fish including northern anchovy, Pacific herring, and smelts (surf and whitebait), may become less abundant in OCNMS (Section 4.8). The abundance of these species is currently highly variable, though. Additionally, migratory fish species, including Pacific hake, jack and Pacific chub mackerel, and Pacific sardine, are likely to become more abundant. Upper trophic-level species, like cetaceans (Section 4.11) and seabirds (Section 4.9), may have some capacity to adapt to these changes by altering their feeding behavior and targeting new or more abundant species. Even in instances in which conditions during El Nino-Southern Oscillation (ENSO; Section 6.3) warm phases, for example, are used to project future climate-related trends, it is not entirely clear that these short-term events are perfect analogs for projected long-term climate trends.
Adapting to climate change within the sanctuary will be shaped in part by the need to decide if, when, and where adaptation should be directed at helping species and ecosystems increase their resistance, resilience, or ability to respond to climate change. In some cases these choices will be made based on what is required to meet the mission of the sanctuary and other directives governing the activities within the sanctuary. How those directives are interpreted may simultaneously be influenced by climate change as well.
There are several tenets or guiding principles related to "climate-smart conservation" that can be used to frame adaptation thinking in the sanctuary. These include protect adequate and appropriate space, manage for uncertainty and expect surprises, reduce non-climate stresses, mainstream climate adaptation, plan for both climate variability and climate change, and reduce the rate and extent of climate change. These guiding principles can be used to frame decisions about adaptive actions related to the four management focal points detailed in the 2011 Management Plan and OCNMS Terms of Designation (water, habitats, living resources, and maritime archaeological resources).
Key Words
Climate Change, Olympic Coast, Olympic Peninsula, Washington Coast, Marine Ecology, Climate Impact, Climate Assessment